
Efficacy of Light and Odor Barriers in Controlling Insect Pest Evasion by Modulating Light and Gas Environments
Ⓒ The Korean Environmental Sciences Society. All rights reserved.
This is an Open-Access article distributed under the terms of the Creative Commons Attribution Non-Commercial License (http://creativecommons.org/licenses/by-nc/3.0) which permits unrestricted non-commercial use, distribution, and reproduction in any medium, provided the original work is properly cited.
Abstract
Insect pests are a significant threat to stored crops and can lead to considerable economic losses and reduced crop quality. Traditional pest control methods often involve chemical treatments, which have adverse environmental and health effects. This study evaluated the effectiveness of controlling the environment using LED lighting and plant-derived odor barriers as a dual strategy for insect pest control. The storage environment was altered using LED lights that emitted specific wavelengths (580–585 nm) and by reducing other wavelengths (300–500 nm). This light environment was combined with an insect odor barrier derived from Cinnamomum verum, Illicium verum, and Artemisia annua, and their duel impact on the behavior and frequency of insect pests under real storage conditions was determined. The findings revealed significant changes in the frequencies of various insect orders, indicating differential responses to light wavelengths and odor barriers. Notably, the introduction of an anti-insect light and odor barrier environment reduced Diptera and Hemiptera frequencies, which could potentially reduce pest intrusion. The results underscore the potential use of integrated light and odor barriers as a noninvasive and environmentally friendly approach to pest management. This study identifies the specific wavelengths and odor combinations that effectively deter insect pests and contribute to the development of more efficient and sustainable pest control methods. It also highlights the importance of understanding insect behavior and frequency changes in response to novel deterrent strategies.
Keywords:
Anti-insect scent, Artificial light at night, LED, Stored product, Stored insect1. Introduction
Some insect species are drawn to light at night due to nocturnal phototaxis, a phenomenon that occurs for various reasons across different species (Park and Lee, 2017; Kim et al., 2019). Interestingly, the exact cause or hypothesis explaining these behavioral traits remains varied and unclear. Theories include the escape mechanism where insects use light as a guide (Mazokhin-Porshnyakov, 1960), navigating by the moon for spreading (Sotthibandhu and Baker, 1979; Juddin et al., 2023), attraction to the thermal energy of light sources (Callahan, 1965; Liu et al., 2022), the sensitivity of insect vision (Robinson and Robinson, 1950; Hsiao, 1973) , and the more recent theory that insects instinctively turn their dorsum toward the light (Fabian et al., 2024).
Nevertheless, humans have utilized insects' nocturnal phototaxis for a long time-approximately 1 A.D; in the Roman Empire, artificial light at night was a method to trap insects (Gardiner, 1995). Even recently, This trait has been applied to protect storages from insets by light traps (Kim et al., 2019). The efficiency of light insect traps is affected by light sources, and the LED (light-emitting diode) technology improves insect trapping (Cohnstaedt et al., 2008; Ramamurthy et al., 2010).
However, sometimes, these technologies that apply the nocturnal phototaxis of insects have significant drawbacks. This disadvantage arises from the fact that selective utilization is not possible. For example, artificial light at night disrupts interaction and changes insect communities, which could devastate some insect species (Deichmann et al., 2021; Grubisic and van Grunsven, 2021). However, lighting at night is necessary for human convenience or to increase work efficiency. Therefore, finding a light source that does not attract or distribute insects is necessary. After that, by adding other anti-insect materials, it would be possible to create an insect barrier system with increased efficiency while minimizing the impact on the ecosystem.
For the anti-insect materials in this study, we used an anti-insect odor barrier from a combination pellet of Cinnamomum verum, Illicium verum, and Artemisia annua. The plant C. verum is widely used in traditional medicines and is an ecologically safe biopesticide (Narayanankutty et al., 2021). Also, I.verum has an insecticidal effect (Aungtikun et al., 2021). And Deb and Kumar(2020) reported that the essential of A. annua showed Artemisia annua holds efficient insecticidal properties against Tribolium casteneum. To add the anti-insect effect to anti-insect light, we will try to create and apply a scent barrier using a mixture of pellets extracted from the three plants with excellent anti-insect effects mentioned above.
In this study, we compare the LED lighting source, named anti-insect light, which primarily emitted 580 nm to 585 nm wavelengths while reducing 300 nm to 500 nm wavelengths than regular LED lighting sources. Also, we testing added an anti-insect odor to the anti-insect light. The first hypothesis is that the anti-insect lighting environment will be the same as the dark one for insects. In addition, the second hypothesis is that adding an anti-insect odor to the light would show an anti-insect effect, at least without a synergy effect at the experimental location simulating actual storage conditions.
2. Materials and Methods
2.1. Experimental location and Insect capturing
The experimental location simulating actual storage conditions was at Sihwa Factory 101, Gongdan 1-aero, Siheung-si, Gyeonggi-do, Republic of Korea. Experiments were conducted at warehouse Site A (33.22 × 3.60 × 3.00 m) and Site B (51.00 × 14.50 × 5.93 m). The distance from the warehouse door to the anti-insect light was 4.77 m and 5.86 m at Site A and Site B, respectively. Test 1 was conducted at Site A from July 17 to August 7, 2023 (21 days). Tests 2 and 3 were conducted at Site A and Site B, respectively, from August 7 to September 12, 2023 (36 days), and Test 4 was conducted at Site B from September 12 to October 12, 2023 (30 days). Insects were captured using a sticky trap A, a 100 × 250 mm yellow sticky trap (Dusong Co., Hanam, Korea), and sticky trap B, a 220 × 330 mm yellow sticky trap (Daegil Co., Gimhae, Korea). Sticky trap A was utilized in Tests 1 and 2, while sticky trap B was employed in Tests 3 and 4.
2.2. Preparation of anti-insect light and anti-insect odor barrier
The LED for the anti-insect light source primarily emitted wavelengths of 580 nm to 585 nm while reducing wavelengths from 300 nm to 500 nm (Fig. 1).
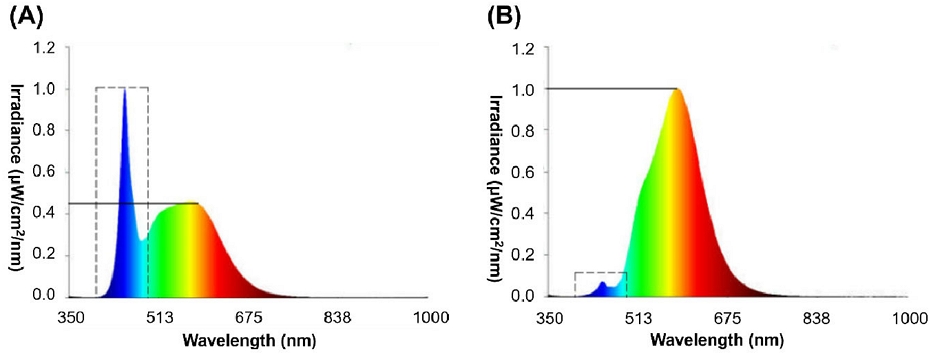
The spectral distribution of the LED light source was used in the test. A is for a regular LED light source, and B is for an anti-insect LED light source.
The anti-insect odor barrier source was produced using C. verum, I. verum, and A. annua (8:1:1, w/w). The mixed plant materials were ground to sizes between 10 μm and 50 μm. The ground plant materials (100 g) were processed in an ultrasonic homogenizer (VC505, Sonics & Materials INC., USA) with ethanol (400 g) for 2 hours. Subsequently, the mixture was stirred at 300 rpm for 24 hours using a magnetic stirrer (BL1003, MTOPS, Korea). The stirred material was then dried under ambient temperature and pressure conditions using a dehydrator (W110, Hanil, Korea) at 300 rpm for 15 days. The dried insect repellent material was aged in a sealed container for approximately 30 days, after which it was processed into pellets for use. The produced pellets were placed in a hemp bag (size 20×30 cm) made of cloth and hung under an LED light source for testing.
2.3. Classification of captured insects
The captured insects were classified through visual inspection and microscopic examination (AM4013MTL, Dino-Lite, Taiwan). A doctoral researcher with over twenty years of experience in insect taxonomy and another researcher with more than ten years of experience cross-validated each insect order.
2.4. Data analysis
Data obtained from image analyses were processed using Microsoft Excel and R software. The sizes of sticky traps used in Tests 1 and 2 differed from those in Tests 3 and 4, as did the duration of insect capture. To standardize these test results, the number of captured insects was divided by the area of the sticky trap, resulting in a measure of captured insects per square meter. This value was further divided by the number of days to yield a rate of insect capture per square meter per day. Organizing the data and performing basic calculations and unit conversions, such as total and 'others' sums, were carried out in Excel. Statistical analysis, specifically the Wilcoxon rank-sum test, was conducted using R software (Wilcoxon, 1945).
3. Results
Comparative tests were conducted to evaluate the effectiveness of anti-insect light. Insects, known for their phototaxis, are typically attracted to light sources. In light of this, our experiment was structured to compare environments treated with anti-insect light against those that were dark and light-free. Owing to limitations in our experimental setup and the time-sensitive nature of these tests, Test 1 and Test 2 were carried out sequentially. Noteworthy was the observation of variations in insect species composition, likely due to the tests being performed in different actual storage conditions.
Table 1 provides a detailed account of the insect orders and their observed frequencies in each test. Test 1 detected nine insect orders (Diptera, Hemiptera, Hymenoptera, Coleoptera, Araneae, Lepidoptera, Thysanoptera, Odonata, and Orthoptera), while Test 2 noted seven insect orders (Diptera, Hemiptera, Hymenoptera, Coleoptera, Araneae, Odonata, and Orthoptera). The frequency and density of each insect order varied between these tests. In Test 1, 40.69 insects/m2/day were captured, making it the highest density observed, while Test 2 recorded the lowest density in this study with 18.48 insects/m2/day. Diptera was the most prevalent in Test 1, but its tendency shifted to Hemiptera in Test 2.
Despite these differences, the findings suggested some efficacy of the anti-insect light. Considering all captured insect species, According to Tables 2 and 3, the p-values obtained from the Wilcoxon rank sum tests exceeded 0.05, indicating that there was no statistically significant difference in insect attraction between environments treated with anti-insect light and those that were kept dark and light-free.

Comparative results of test 1: Analysis of the efficacy of anti-insect light treatment versus dark conditions using the Wilcoxon rank sum test

Comparative results of test 2: Analysis of the efficacy of anti-insect light treatment versus dark conditions using the Wilcoxon rank sum test
To further test efficacy, an anti-insect scent was added, and Tests 3 and 4 were sequentially conducted under similar experimental constraints. Changes in insect species composition were again observed, attributed to varying real storage conditions.
The observed insect orders and frequencies are outlined in Table 1. Test 3 identified 10 insect orders (Diptera, Hemiptera, Hymenoptera, Coleoptera, Araneae, Lepidoptera, Orthoptera, Psocoptera, Metastigmata, and Ephemeroptera), whereas Test 4 noted 7 (Diptera, Hemiptera, Hymenoptera, Coleoptera, Araneae, Lepidoptera, and Ephemeroptera). The frequency and density of each insect order varied, with Test 3 recording the highest density of the insects (45.71 captured insects/m2/day). Test 4 had 32.22 captured insects/m2/day, with Diptera and Hemiptera being the most frequent but less dense than in Test 3.
These results indicate that the combination of anti-insect light and scent was effective. Table 4 showcases the densest insect captures from Test 3. Excluding the number of minor capturing insects order, the numbers of Diptera, Hemiptera, Hymenoptera, and their sum of all captured insects were significantly different from the control, with p-values from the Wilcoxon rank sum test below 0.001. Test 4 results, presented in Table 5, test 4 showed a similar composition to test 3 but with lower density. The total insect count and Hemiptera numbers were significantly different from the control, while numbers for Diptera, Hemiptera, and other captured insects showed no significant differences.

Comparative results of test 3: Evaluating anti-insect light treatment combined with natural scent barrier against dark conditions through Wilcoxon rank sum test
4. Discussion
In this study, we tested the LED lighting source that primarily emitted 580 nm to 585 nm wavelengths while reducing 300 nm to 500 nm wavelengths compared with regular LED lighting sources. The result of this environmental control showed that our first hypothesis -the anti-insect lighting environment will be the same as dark for insects- is correct. Additionally, our results are supported by recent studies, such as Liu et al.(2018), which demonstrated a phototaxis preference rank in Bactrocera dorsalis Hendel (Diptera: Tephritidae) is red (633 nm), blue (450 nm), purple (440 nm), yellow (596 nm), and green (522 nm). Similarly, the compact fluorescent, which emits mainly around 550 nm and 600 nm to 650 nm wavelengths, has fewer disturbances to wildlife (Wakefield et al., 2016).
The anti-insect odor also showed an anti-insect effect. Also, despite the limited experimental environment of the experimental location simulating actual storage conditions, it is predicted that there will be a synergy effect between anti-insect light and anti-insect odor.
Summarizing the experimental results, comparing the dark conditions and the anti-insect light treatment environment in tests 1 and 2 showed no statistical difference in the number of insects collected between the control and treatment groups (Table 2, 3). Despite the differences in sites (Site A and Site B) and collection times, the results indicate that the anti-insect light treatment did not attract insects. Although this study lacks experiments specifically testing insect attraction to different light conditions, general research demonstrates the effects of light wavelength on insect attraction (Liu et al., 2018). In contrast, insects were repelled when the odor barrier effect was added in the same environment (Table 4, 5). This effect was consistent across different sites and collection times. Notably, the effect was pronounced at the time point of test 3, when insect density was high, and it showed statistically significant effects on Hemiptera during test 4, which had a lower insect density. This suggests that the effectiveness of the treatment might be higher when insect density is elevated.
Future research should focus on validating the synergistic effects of anti-insect light combined with anti-insect odor treatment in authentic storage conditions to enhance pest control strategies.
REFERENCES
-
Aungtikun, J., Soonwera, M., Sittichok, S., 2021, Insecticidal synergy of essential oils from Cymbopogon citratus (Stapf.), Myristica fragrans (Houtt.), and Illicium verum Hook. f. and their major active constituents, Ind. Crop. Prod., 164, 113386.
[https://doi.org/10.1016/j.indcrop.2021.113386]
-
Callahan, P., 1965, Far infra-red emission and detection by night-flying moths, Nature, 206, 1172-1173.
[https://doi.org/10.1038/2061172a0]
-
Cohnstaedt, L., Gillen, J. I., Munstermann, L. E., 2008, Light-emitting diode technology improves insect trapping, JAMCA, 24, 331.
[https://doi.org/10.2987/5619.1]
-
Deb, M., Kumar, D., 2020, Bioactivity and efficacy of essential oils extracted from Artemisia annua against Tribolium casteneum (Herbst. 1797) (Coleoptera: Tenebrionidae): An Eco-friendly approach, Ecotoxicol. Environ. Saf., 189, 109988.
[https://doi.org/10.1016/j.ecoenv.2019.109988]
-
Deichmann, J. L., Ampudia Gatty, C., Andía Navarro, J. M., Alonso, A., Linares‐Palomino, R., Longcore, T., 2021, Reducing the blue spectrum of artificial light at night minimises insect attraction in a tropical lowland forest, Insect Conserv. Diver., 14, 247-259.
[https://doi.org/10.1111/icad.12479]
-
Fabian, S. T., Sondhi, Y., Allen, P. E., Theobald, J. C., Lin, H. T., 2024, Why flying insects gather at artificial light, Nat. Commun., 15, 689.
[https://doi.org/10.1038/s41467-024-44785-3]
- Gardiner, B., 1995, The very first light-trap, 1565, Ent. Rec. J. Var., 107, 45-46.
-
Grubisic, M., van Grunsven, R. H., 2021, Artificial light at night disrupts species interactions and changes insect communities, Curr. Opin. Insect Sci., 47, 136-141.
[https://doi.org/10.1016/j.cois.2021.06.007]
-
Hsiao, H. S., 1973, Flight paths of night-flying moths to light, J. Insect Physiol., 19, 1971-1976.
[https://doi.org/10.1016/0022-1910(73)90191-1]
-
Juddin, A. S. S., Ngah, N., Umar, R., Asante, K., Abdullahi, M. G., 2023, A Review influence of light on insect activity and behaviour: Sustainable lighting and light pollution, JSSM, 18, 231-247.
[https://doi.org/10.46754/jssm.2023.02.015]
-
Kim, K. N., Huang, Q. Y., Lei, C. L., 2019, Advances in insect phototaxis and application to pest management: A Review, Pest Manag. Sci., 75, 3135-3143.
[https://doi.org/10.1002/ps.5536]
-
Liu, H., Gao, Z., Deng, S. Z., Cao, F. Q., Lu, Y. Y., 2018, The photokinesis of oriental fruit flies, B. actrocera dorsalis, to LED lights of various wavelengths, Entomol. Exp. Appl., 166, 102-112.
[https://doi.org/10.1111/eea.12648]
-
Liu, Q. H., Zhao, M. F., Wu, Y. Q., 2022, Effects of Spectral Light Factors on the Phototactic Response of Frankliniella occidentalis (Thysanoptera: Thripidae) Using LED Source, J. Nanoelectron. Optoelectron., 17, 1299-1307.
[https://doi.org/10.1166/jno.2022.3298]
- Mazokhin-Porshnyakov, G., 1960, Why insects fly to light by night, Revue d’Entomologie de l’URSS, 39, 52-58.
-
Narayanankutty, A., Kunnath, K., Alfarhan, A., Rajagopal, R., Ramesh, V., 2021, Chemical composition of Cinnamomum verum leaf and flower essential oils and analysis of their antibacterial, insecticidal, and larvicidal properties, Molecules, 26, 6303.
[https://doi.org/10.3390/molecules26206303]
-
Park, J. H., Lee, H. S., 2017, Phototactic behavioral response of agricultural insects and stored-product insects to light-emitting diodes (LEDs), App. Biol. Chem., 60, 137-144.
[https://doi.org/10.1007/s13765-017-0263-2]
- Ramamurthy, V. V., Akhtar, M. S., Patankar, N. V., Menon, P., Kumar, R., Singh, S. K., Ayri, S., Parveen, S., Mittal, V., 2010, Efficiency of different light sources in light traps in monitoring insect diversity, Mun. Ent. Zool., 5, 109-114.
- Robinson, H., Robinson, P., 1950, Some notes on the observed behaviour of Lepidoptera in flight in the vicinity of light-sources together with a description of a light-trap designed to take entomological samples, Entomologist’s Gazette, 1, 3-15.
-
Sotthibandhu, S., Baker, R., 1979, Celestial orientation by the large yellow underwing moth, Noctua pronuba L, Anim. Behav., 27, 786-800.
[https://doi.org/10.1016/0003-3472(79)90015-0]
-
Wakefield, A., Broyles, M., Stone, E. L., Jones, G., Harris, S., 2016, Experimentally comparing the attractiveness of domestic lights to insects: Do LED s attract fewer insects than conventional light types?, Eco. Evo., 6, 8028-8036.
[https://doi.org/10.1002/ece3.2527]
-
Wilcoxon, F., 1945, Individual comparisons by ranking methods, Biometrics Bull., 1, 80–83.
[https://doi.org/10.2307/3001968]
Institute of Life Science and Natural Resources, Korea University rhacoy@korea.ac.kr
Sihwa Factory 101, SPC SAMLIP Co., ltd. mold95@spc.co.kr
R&D Center, DSE Co., ltd. kwonyh@outlook.com
HIPOS R&C Co., Ltd., Korea University acgcorn@korea.ac.kr
Biology and Agriculture Lab., Department of Practical Arts Education, Cheongju National University of Education hangds@cje.ac.kr