
Influence of Environmental Conditions on the Growth and Biochemical Composition of Azolla imbricata (Roxb.) Nakai, a Paddy Field Biofertilizer in Korea
Ⓒ The Korean Environmental Sciences Society. All rights reserved.
This is an Open-Access article distributed under the terms of the Creative Commons Attribution Non-Commercial License (http://creativecommons.org/licenses/by-nc/3.0) which permits unrestricted non-commercial use, distribution, and reproduction in any medium, provided the original work is properly cited.
Abstract
Plants face various challenges owing to seasonal variations, particularly Azolla imbricata, a potent biofertilizer in paddy fields that significantly contributes to rice yields. In Korea, temperature (T) and humidity (H) fluctuate substantially between planting and harvesting paddy fields. Therefore, this study investigated the effects of the distinct T and H conditions of Korea, both with (nutrient solution, NS) and without (water media, WM) nutrients, on the growth, photosynthetic pigments, and plant stress markers, such as total flavonoid (TFC) and phenolic contents (TPC) of A. imbricata. Regardless of nutrient availability, growth thrived under 25°C T and 60% H conditions. Comparatively, biomass production was more robust in NS than in WM. Furthermore, a correlation analysis of the growth data developed during NS and WM exposure revealed that biomass was positively influenced by T and negatively affected by H. Photosynthetic pigments, namely chlorophyll a, b, and a+b, suggested that the tolerance mechanism of A. imbricata may aid in its survival under a diverse array of T/H conditions, even when nutrients are absent. The accumulation of TFC and TPC demonstrated that A. imbricata made considerable efforts to withstand these stresses independent of T and H. In conclusion, this study reveals that the optimal growth of A. imbricata occurs at 25°C T and 60% H conditions, which may facilitate increased rice yields. Additionally, nutrients can be supplemented in paddy fields to enhance the rice yield under the remaining environmental conditions.
Keywords:
Azolla, Biomass, Photosynthetic pigments, Temperature, Humidity1. Introduction
Seasonal variations are closely related to the chemical composition and bioactivity of plants. Testing secondary metabolites is a way to determine a plant’s resistance to these variations. During the stress, plant activate various survival mechanisms, particularly the biosynthesis and accumulation of secondary metabolites such as phenolic and flavonoid contents (Park et al., 2023). Phenolic and flavonoid compounds are important secondary metabolites that plants release in response to biotic and abiotic stresses like pathogen and insect attacks, drought, UV radiation, and extreme or low temperatures (T) and humidity (H). Phenolic compounds are essential for plant survival and are modified in response to environmental disturbances through various physiological functions (Song and Choi, 2017) such as isoprenoid, phenylpropanoid, alkaloid, and fatty acid pathways (Kumar et al., 2023). Similarly, phenolic compounds also serve as antioxidants, protecting plants from oxidative stress and harmful reactive oxygen species (Hithamani et al., 2022). Moreover, a sign that plants are growing properly is their high concentration of photosynthetic pigments such as chlorophyll and carotenoids. The literature also records that external stress can result in the reduction of these photosynthetic pigments (Amin et al., 2024).
In general, the seasonal temperature (‘T’) and humidity (‘H’) fluctuations are significant in high-temperature regions. As a result, plants in these areas frequently encounter these stresses. Although, through evolution, plants have naturally developed adaptive abilities to resist these stresses, organisms that depend on these plants are facing a serious problem. This naturally occurring adverse phenomenon also applies to agricultural lands. Considering temperature and humidity, either individually or together, numerous studies have proven that both high and low levels of these factors can influence plant growth (Shi et al., 2022).
Plants fix atmospheric nitrogen through their symbiotic relationships with soil or rhizobial bacteria. However, water flooding up to 10 cm above the soil surface in paddy fields may inhibit nitrogen fixation by soil bacteria. Therefore, the rapidly growing floating fern Azolla, a biofertilizer that doubles its biomass in 10 days, is introduced to paddy fields to fix atmospheric nitrogen. Their specialized leaf cavities serve as the habitat for the cyanobacterium Anabaena azollae, which fixes atmospheric nitrogen and supplies it to Azolla (Abd El-Aal, 2022). At the end of the Azolla life cycle, its dead and decaying tissues provide ample amounts of stored nitrogen to rice plants (Rascio and La Rocca, 2018). Further, many studies have been conducted around the world on the effect of temperature and humidity on various plant species other than Azolla (Hoang and Kim, 2018). Meanwhile, Azolla is introduced into South Korean paddy fields during May–June and September–October (around 5 months), marking the period between the plantation and harvest of rice. Naturally, during this period, Azolla in rice fields is exposed to extreme variations in temperature (between 16°C and 25°C) and humidity (between 64% and 80%) (https://www.weather-atlas.com/). The seasonal variation in temperature and humidity in South Asian countries are very low, therefore, detailed studies on the effects of temperature and humidity on the growth of Azolla have not been conducted (Nam and Yoon, 2008). Therefore, this study takes this into account. Further, to our knowledge, there are no studies that comprehensively evaluate the growth and biochemical parameters such as biomass production, photosynthetic pigments (chlorophyll, and carotenoids), total phenolic (TPC), and flavonoid (TFC) contents of Azolla under different Korean environmentally relevant conditions such as temperature and humidity. We tested the effects of various temperature and humidity conditions related to the South Korean climate on the growth of Azolla along with nutrient stress. Through this study’s results, we can identify the period when Azolla growth slows down, thereby increasing rice production by using more nitrogen fertilizers.
2. Materials and Methods
2.1. Cultivation of Azolla
The aquatic fern, Azolla imbricata (Roxb.) Nakai was grown in a greenhouse for 3 years using a nitrogen-free nutrient medium. The NS was refreshed every second week.
2.2. Experimental design
Deionized water (WM) or nutrient solution (NS) amounting to 250 ml was added to transparent plastic containers (17×12×5.5 cm3). These containers were then covered with black paper tape to prevent light penetration from the sides. Subsequently, the containers were moved to the plant growth chamber (LabTech, LGC-4201, South Korea). An experiment, utilizing a light and dark regime of 16 h (2200 lx) and 8 h respectively, was conducted. The minimum, mean, and maximum temperature (T) (20, 25, and 30°C) and humidity (H) (60, 70, and 80%) conditions, representative of Korean paddy fields, were selected for this study. Based on these temperature (T) (°C) and humidity (H) (%) ranges, the following experimental condition combinations (T/H) were chosen: 20°C/70%, 25°C/70%, 30°C/70%, 30°C/80%, 25°C/60%, and 25°C/80%.
Well-grown A. imbricata was collected from the culture and washed several times with deionized water. Approximately 1 g of freshly weighed Azolla, surface-dried with Kleenex dry cell towels, was then placed in each container. The evaporated solution in each container was compensated with deionized water every 3 days until the end of the experiment. On the final day, Azolla was separated from the medium, washed several times again with deionized water, and dried with Kleenex dry cell towels. The Azolla was then immediately used for the analysis of biomass, photosynthetic pigments (chlorophyll a, b and a+b), and total phenolic (TPC) and flavonoid contents (TFC). All experiments were replicated three times.
2.3. Estimation of biomass
After the experiment, the percentage increase or decrease in biomass was calculated using the following equation:
2.4. Estimation of chlorophyll and carotenoid contents
To extract chlorophyll (a, b, and total) and carotenoids, we mixed 1 g of fresh Azolla with 10 mL of methanol. We covered these tubes with lids and incubated them in the dark for 24 h. After incubation, we measured the absorbance of the supernatant at 470 nm, 652 nm, and 665 nm. We calculated the concentrations of photosynthetic pigments (µg/mL) using the following equations:
2.5. Estimation of total flavonoid and phenolic contents
The total flavonoid content (TFC) and phenolic content (TPC) were measured as per the method proposed by Shekhar et al.(2015). Briefly, TFC and TPC were measured by grinding 0.25 g of A. imbricata in 80% aqueous methanol (1:10 ratio) and shaking at 150 rpm for 1 h at 25°C. The alcoholic extract was then centrifuged and used for TPC and TFC analysis. For TFC, the supernatant was diluted with 5 ml of Milli-Q water and 0.3 ml of 10% NaNO2. The samples were then incubated at 25°C for 5 min and further incubated for 10 min following the addition of 0.3 ml of 10% AlCl3 and 2 ml of 1 N NaOH. The absorbance was finally read at 510 nm using Milli-Q water as a blank. For TPC, the supernatant was diluted with 1 ml of Milli-Q water, 5 ml of 10% Folin-Ciocalteu reagent, and 4 ml of 7% Na2CO3. The samples were then incubated for 30 min and the absorbance was read at 760 nm. TFC was expressed in milligrams of quercetin equivalent (QE)/100 g, while TPC was expressed in mg of gallic acid equivalent (GAE)/100 g.
2.6. Data analysis
For all parameters, Duncan’s multiple range test (DMRT, P < 0.05) was utilized to compare results under various T/H conditions. A two-way analysis of variance (ANOVA) was employed to assess the significant (P < 0.05) impact of individual variables (T/H and media), in addition to their combined effect on all parameters tested. A two-tailed Pearson correlation analysis was conducted to evaluate associations between the experimental parameters (T, H, and media) and all tested parameters. All tests were conducted using SPSS version 25.
3. Results
3.1. Biomass of A. imbricata
Azolla, initially exposed to one gram, demonstrated a weight gain in all experiments after 10 days (Fig. 1). The percentage of biomass increase was high (195%) and low (36%) in temperature/humidity condition of 25°C/60% and 25°C/70% in WM, respectively. In the case of NS, the percentage of biomass increase was high (640%) and low (155%) in temperature/humidity condition of 25°C/60% and 25°C/80%, respectively (DMRT, P < 0.05). Comparing WM and NS, all experimental conditions (T/H) revealed that biomass production in NS was 2.3 at 20°C/70% and 30°C/70% to 6.2 at 25°C/60% times higher than in WM (Fig. 1). Furthermore, two-way ANOVA indicated that the biomass was significantly impacted by the tested experimental conditions (T/H) and media (NS and WM) (P < 0.05) (Table 1).
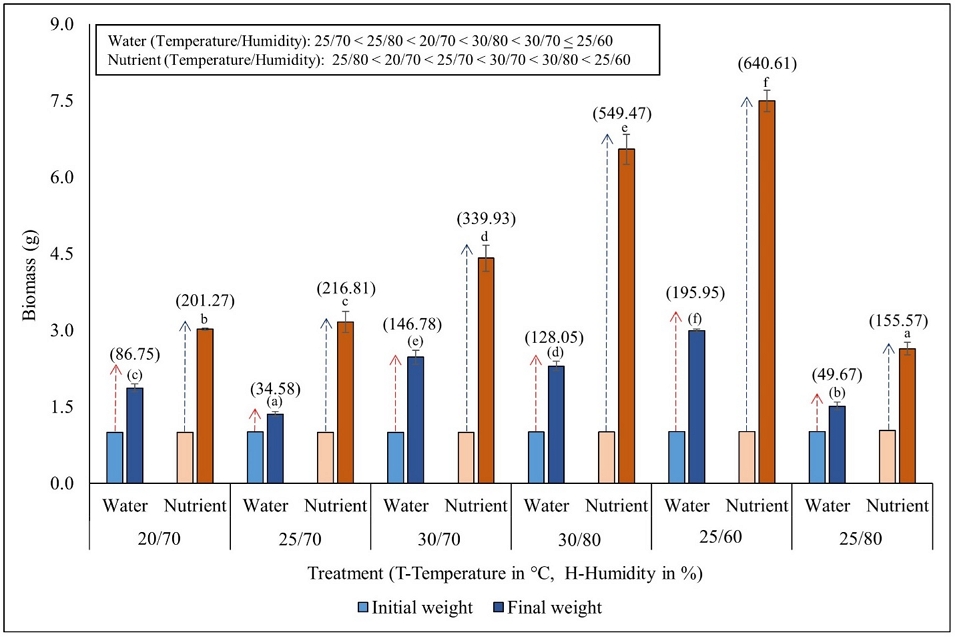
Effect of environmental conditions (temperature, °C/humidity, %) on the biomass production of Azolla imbricata growing in water and in nutrient media. Numerical values within brackets above the error bars indicate the percent increase in biomass after 10 days of the experiment. Different alphabetical characters above the error bars (values within brackets compared for water media and without brackets compared for nutrient media) indicate significant (DMRT, P<0.05) differences among the temperature conditions.
3.2. Chlorophyll and carotenoid content of A. imbricata
The minimum (37 µg/ml) and maximum (48.8 µg/ml) values of chlorophyll a was found in temperature/humidity conditions of 20°C/70% and 25°C/60% in WM. Similarly, in NS, the minimum (34.8 µg/ml) and maximum (50.4 µg/ml) values were found in temperature/humidity conditions of 20°C/70% and 25°C/60% (Fig. 2a). The minimum and maximum values of chlorophyll b in WM and NS were 13.2 µg/ml in 30°C/70% and 31.9 µg/ml in 20°C/70%, and 8.7 µg/ml in 25°C/60% and 19.6 µg/ml in 20°C/70%, respectively (Fig. 2b). The minimum, and maximum values of total chlorophyll (a+b) in WM and NS were 52.2 µg/ml in 30°C/80% and 69.4 µg/ml in 20°C/70% and 54.4 µg/ml in 20/70 and 60.6 µg/ml in 25/70, respectively (Fig. 2c). Similarly, the minimum and maximum values of carotenoid content in WM and NS were 3576 µg/ml in 25°C/70% and 4785 µg/ml in 25°C/80%, and 3528 µg/ml in 20°C/70% and 4397 µg/ml in 25°C/60%, respectively (Fig. 3). The pigment values differed significantly among the experimental conditions (T/H; DMRT, P < 0.05). A two-way ANOVA revealed that all pigment contents were significantly impacted by environmental conditions (T/H) and media (WM and NS) (P < 0.05; Table 1).
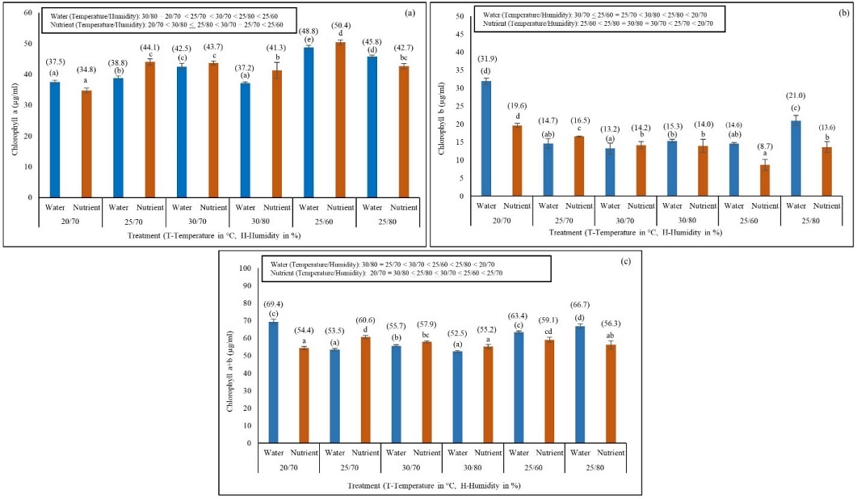
Effects of different environmental conditions (temperature, °C/humidity, %) on chlorophyll a (a), b (b), and a+b (c) content (µg/ml) of Azolla imbricata growing in water and nutrient media. Chlorophyll values after 10 days of the experiment are indicated within brackets above the error bars. Different alphabetical characters above the error bars (values within brackets compared for water media and without brackets compared for nutrient media) indicate significant (DMRT, P<0.05) differences among the temperature conditions.
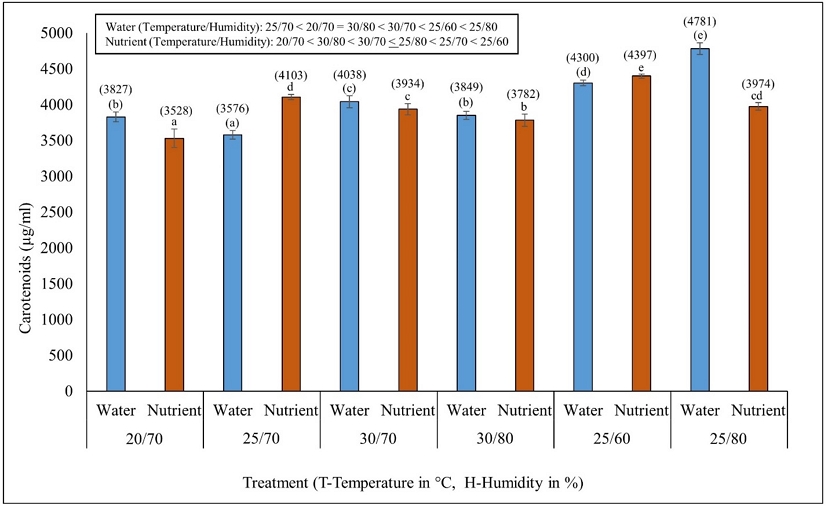
Effect of different environmental conditions (temperature, °C/humidity, %) on the carotenoid content (µg/ml) of Azolla imbricata growing in water and nutrient media. Carotenoid content after 10 days of the experiment is indicated within brackets above the error bars. Different alphabetical characters above the error bars (values within brackets compared for water media and without brackets compared for nutrient media) indicate significant differences (DMRT, P<0.05) among the temperature conditions.
3.3. Total flavonoid and phenolic contents of A. imbricata
The TFC and TPC significantly differed between the experimental conditions (Fig. 4). The maximum and minimum value of TFC was 664 QE mg/100 g in 25°C/80% and 140 QE mg/100 g in 25°C/70% in WM. Further, in NS, the minimum was 273 QE mg/100 mg and maximum, was 664 QE mg/100 g values of TFC was found in 25°C/70% and 25°C/80%, respectively. Likewise, the minimum and maximum values of TPC was 19 GAE mg/100 g in 25°C/80% and 32 GAE mg/100 g in 25°C/60% conditions in WM, and 13 GAE mg/100 g in 25°C/80% and 52 GAE mg/100 g in 25°C/60% conditions in NS. Two-way ANOVA revealed that TPC and TFC were significantly affected by environmental conditions (T/H) and media (WM and NS) (P < 0.05; Table 1), except for the influence of media alone on TPC.
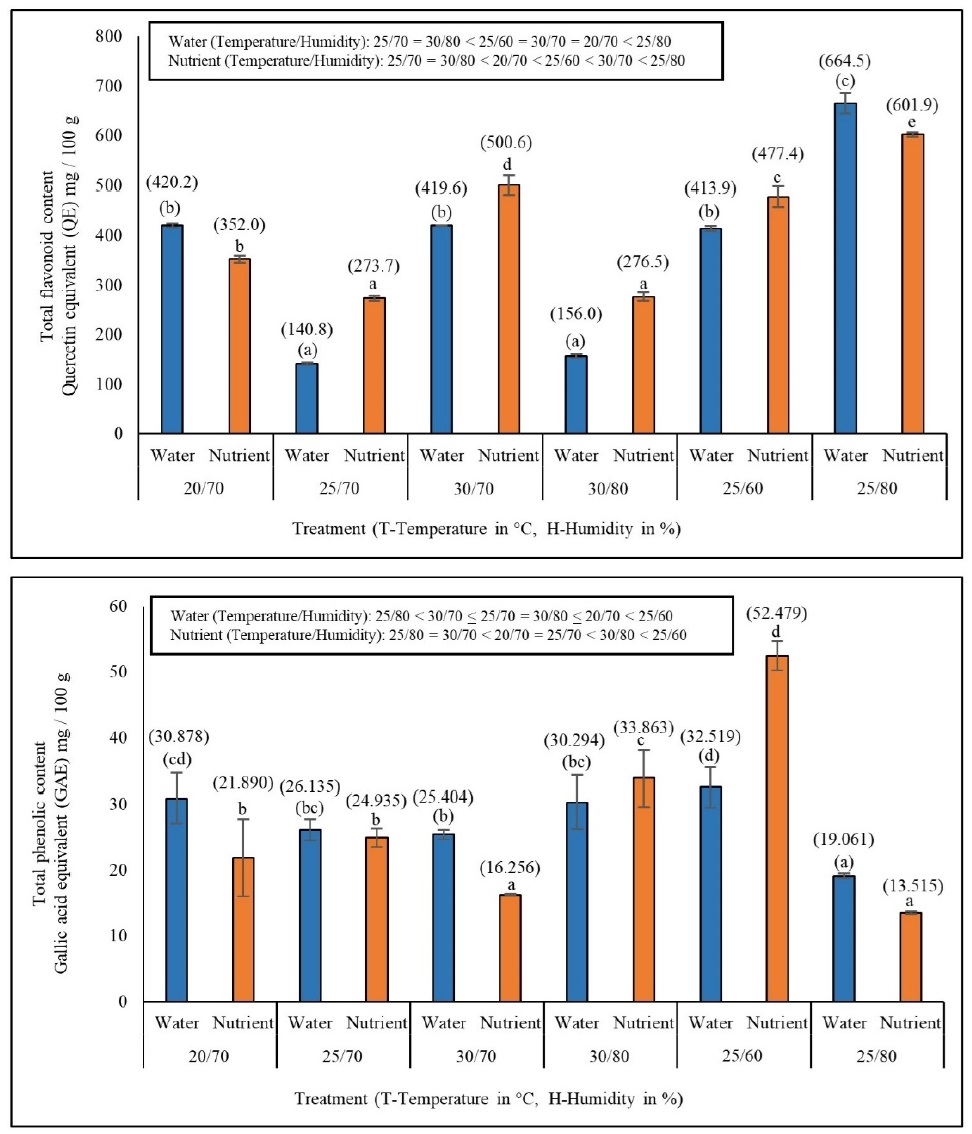
Effect of different environmental conditions (temperature, °C/humidity, %) on total flavonoid (a) and phenolic (b) content of Azolla imbricata growing in water and nutrient media. Total flavonoid and phenolic contents after 10 days of the experiment are indicated within brackets above the error bars. Different alphabetical characters above the error bars (values within brackets compared for water media and without brackets compared for nutrient media) indicate significant differences (DMRT, P<0.05) among the temperature conditions.
4. Discussion
Previous studies have demonstrated that the biomass production of Azolla is suppressed in the presence of inappropriate nutrient ratios in media, and confounding environmental conditions such as extreme temperatures and humidity levels (Sadeghi et al., 2013; Hossain et al., 2021; da Silva et al., 2022). The results of the current study validate these findings. In this study, we found that biomass production in the NS was significantly higher (as indicated by a two-way ANOVA, P < 0.05) than in the WM in all temperature/humidity (T/H) experiments. Regardless of nutrient availability (NS vs. WM), biomass at 25°C with 60% humidity surpasse that of other environmental conditions. Moreover, biomass in NS exceeded that in WM by a factor of 3.2. This boost in biomass production in NS can be attributed to the presence of a balanced nutrient ratio, promoting a high rate of metabolic chemical reactions within cells and aiding in Azolla growth.
In the face of varying nutrient conditions, our results propose that temperatures above 25°C and humidity either below or above 60% can detrimentally impact A. imbricata growth. This finding was corroborated by a two-way ANOVA (P < 0.05), as the A. imbricata biomass was shown to be significantly influenced by temperature, humidity, and media (WM and NS), all environmentally relevant conditions in Korea (Table 1). Therefore, based on our findings, we can hypothesize that a temperature of 25°C and a humidity level of 60% provide optimal conditions for A. imbricata growth.
The growth of Azolla has a direct relationship with its photosynthetic pigments, such as chlorophyll a, b, and a+b. High levels of these photosynthetic pigments indicate good A. imbricata growth. Though there is significant variation in photosynthetic pigments in A. imbricata across temperature and humidity ranges, similarities in these pigments are found under specific T/H conditions. For example, 30°C/80% is equal to 20°C/70% in WM and 30°C/70% is equal to 25°C/70% in NS for chlorophyll a, 25°C/60% is equal to 25°C/70% in WM, while 25°C/80%, 30°C/80%, and 30°C/70% are equivalent in NS for chlorophyll b. For chlorophyll a+b, 30°C/80% is equal to 25°C/70% in WM, whereas 20°C/70% is equal to 30°C/80% in NS, and for carotenoid contents, 20°C/70% is equal to 30°C/80% in WM, while in NS, 30°C/70% is less than 25°C/80%. These findings suggest that A. imbricata’s tolerance mechanism might enable it to thrive in various T/H conditions, even when nutrients are not readily available (as in WM). Moreover, higher levels of chlorophyll a compared to chlorophyll b in all experiments highlight the potential role of chlorophyll a in minimizing T/H stress.
High TFC and TPC levels in plants indicate external stress (Masood et al., 2006; Nham Tran et al., 2020). In the case of TFC and TPC in this study, both in WM and NS, many T/H conditions were found to have no significant difference. For example, with regards to TFC, the conditions 25°C/70%=30°C/80% and 25°C/60%=30°C/70%= 20°C/70% in WM, and 25°C/70%=30°C/80% in NS were similar. Likewise, for TPC, 25°C/70%=30°C/80% in WM and 25°C/80%= 30°C/70% and 20°C/70%=25°C/70% in NS were also similar. Our current study found significant variances in both TPC and TFC contents (DMRT, P < 0.05) across different environmental conditions. Additionally, a substantial correlation between temperature and humidity with the medium was evidenced by the two-way ANOVA (P<0.05). However, no consistent pattern was observed in both TFC and TPC when considering temperature or humidity alone (Fig. 4). Thus, regardless of temperature and humidity, A. imbricata demonstrates a variety of responses to manage these stresses.
In correlation analysis, regardless of whether nutrients are available, a positive correlation between biomass and temperature (Table 2), and a negative correlation between biomass and humidity (Table 3), suggest that biomass was promoted by the temperature and inhibited by the humidity. This is consistent with previous results showing the effect of high and low temperatures and high humidity on the growth of Azolla (Bocchi and Malgioglio, 2010; Sadeghi et al., 2012; Vafaei et al., 2012). Without nutrients (WM), all photosynthetic pigments (excluding chlorophyll a) and TPC and TFC were negatively impacted by temperature (Table 2). However, this situation changed with the introduction of nutrients (NS), where the correlation was positive, except for chlorophyll b. Humidity consistently exerted negative impacts on biochemical parameters, regardless of nutrient availability, except for carotenoid content and TFC. For WM, biomass had a negative correlation with chlorophyll b and a+b, while only with chlorophyll b in NS, indicating the influence of nutrient availability on photosynthetic pigments. Furthermore, a positive correlation of biomass with TFC in WM turned negative with the addition of NS, underscoring the beneficial role of nutrients in alleviating temperature and humidity stress. However, in both cases (WM and NS), TPC positively correlated with biomass, suggesting that nutrient availability did not affect TPC accumulation in Azolla cells. A more detailed study is needed to validate this statement.

Correlation analysis between temperature, humidity, biomass production and biochemical parameters after 10 days of experiment in water media

Correlation analysis between temperature, humidity, biomass production and biochemical parameters after 10 days of experiment in nutrient solution
The results of the present study confirm that Azolla can tolerate all temperature and humidity (T/H) conditions. However, its growth is optimal at 25°C and 70% humidity. Therefore, it is undeniable that Azolla can enhance rice yield under these specific conditions. For less favourable T/H conditions, where Azolla exhibits slower growth, alternative fertilizers can be utilized to boost rice yield. Insights from this study help identify periods of slowed Azolla growth, allowing for improved rice production via increased use of nitrogen fertilizers.
Acknowledgments
This work was supported by a 2-Year Research Grant of Pusan National University.
REFERENCES
-
Abd El-Aal, A. A. M., 2022, Anabaena-azollae, significance and agriculture application: A case study for symbiotic cyanobacterium, in: Singh, R.P., Bhattacharjee, K., Manchanda, G., Panosyan, H. (Eds.), Microbial Syntrophy-Mediated Eco-Enterprising, Academic Press, 1–14
[https://doi.org/10.1016/b978-0-323-99900-7.00006-7]
-
Amin, B., Atif, M. J., Kandegama, W., Nasar, J., Alam, P., Fang, Z., Cheng, Z., 2024, Low temperature and high humidity affect dynamics of chlorophyll biosynthesis and secondary metabolites in Cucumber, BMC plant biology 24, 903
[https://doi.org/10.1186/s12870-024-05615-2]
-
Bocchi, S., Malgioglio, A., 2010, Azolla-anabaena as a biofertilizer for rice paddy fields in the Po valley, A Temperate rice area in Northern Italy, International Journal of Agronomy
[https://doi.org/10.1155/2010/152158]
-
da Silva, M. E. J., Mathe, L. O. J., van Rooyen, I. L., Brink, H. G., Nicol, W., 2022, Optimal growth conditions for Azolla pinnata R. Brown: Impacts of light intensity, nitrogen addition, pH control, and humidity, Plants, 11, 1048
[https://doi.org/10.3390/plants11081048]
-
Hithamani, G., Naveen, J., Pushpalatha, H. G., 2022, Phenolic compounds: Stress markers of plants and their protective role, Plant Metabolites under Environmental Stress, 249-276
[https://doi.org/10.1201/9781003304869-12]
-
Hoang, L. H. N., Kim, W. S., 2018, Air temperature and humidity affect petunia ornamental value, Horticultural Science and Technology, 36, 10–19
[https://doi.org/10.12972/kjhst.20180002]
-
Hossain, M., Shimu, S., Sarker, M., Ahsan, M., Banu, M., 2021, Biomass growth and composition of Azolla (Azolla Pinnata R. BR.) supplemented with inorganic phosphorus in outdoor culture, SAARC Journal of Agriculture, 19, 177–184
[https://doi.org/10.3329/sja.v19i1.54788]
-
Kumar, K., Debnath, P., Singh, S., Kumar, N., 2023, An Overview of plant phenolics and their involvement in abiotic stress tolerance, Stresses, 3, 570-585
[https://doi.org/10.3390/stresses3030040]
-
Masood, A., Shah, N. A., Zeeshan, M., Abraham, G., 2006, Differential response of antioxidant enzymes to salinity stress in two varieties of Azolla (Azolla pinnata and Azolla filiculoides), Environmental and Experimental Botany, 58, 216–222
[https://doi.org/10.1016/j.envexpbot.2005.08.002]
- Nam, K. W., Yoon, D. H., 2008, Usage of Azolla spp. as a biofertilizer on the environmental-friendly agricultue, Proceedings of the Plant Resources Society of Korea Conference, 5–13.
-
Nham Tran, T. L., Miranda, A. F., Abeynayake, S. W., Mouradov, A., 2020, Differential production of phenolics, lipids, carbohydrates and proteins in stressed and unstressed aquatic plants, azolla filiculoides and azolla pinnata, Biology, 9, 1–15
[https://doi.org/10.3390/biology9100342]
-
Park, Y. J., Kwon, D. Y., Koo, S. Y., Truong, T. Q., Hong, S. C., Choi, J., Moon, J., Kim, S. M., 2023, Identification of drought-responsive phenolic compounds and their biosynthetic regulation under drought stress in Ligularia fischeri, Frontiers in Plant Science, 14
[https://doi.org/10.3389/fpls.2023.1140509]
-
Rascio, N., La Rocca, N., 2018, Biological nitrogen fixation, Encyclopedia of Ecology, 264–279
[https://doi.org/10.1016/B978-0-444-63768-0.00685-5]
- Sadeghi, R., Zarkami, R., Sabetraftar, K., Van Damme, P., 2013, A Review of some ecological factors affecting the growth of Azolla spp., CJES (Caspian J. Env. Sci.), 11, 65–76.
-
Sadeghi, R., Zarkami, R., Sabetraftar, K., Van Damme, P., 2012, Use of support vector machines (SVMs) to predict distribution of an invasive water fern Azolla filiculoides (Lam.) in Anzali wetland, southern Caspian Sea, Iran, Ecological Modelling, 244, 117–126
[https://doi.org/10.1016/j.ecolmodel.2012.06.029]
-
Shekhar, S., Mishra, D., Buragohain, A. K., Chakraborty, S., Chakraborty, N., 2015, Comparative analysis of phytochemicals and nutrient availability in two contrasting cultivars of sweet potato (Ipomoea batatas L.), Food Chemistry
[https://doi.org/10.1016/j.foodchem.2014.09.172]
-
Shi, Y. S., Yang, L., Yu, M. F., Li, Z. H., Ke, Z. J., Qian, X. H., Ruan, X., He, L. P., Wei, F., Zhao, Y. X., Wang, Q., 2022, Seasonal variation influences flavonoid biosynthesis path and content, and antioxidantactivity of metabolites in Tetrastigma hemsleyanum Diels & Gilg, PLoS ONE.
[https://doi.org/10.1371/journal.pone.0265954]
-
Song, W. Y., Choi, J. H., 2017, Total phenols, flavonoid contents, and antioxidant activity of spirodela polyrhiza extracts, Journal of Life Science
[https://doi.org/10.5352/jls.2017.27.2.180]
-
Vafaei, F., Khataee, A. R., Movafeghi, A., Salehi Lisar, S. Y., Zarei, M., 2012, Bioremoval of an azo dye by Azolla filiculoides: Study of growth, photosynthetic pigments and antioxidant enzymes status, International Biodeterioration and Biodegradation, 75, 194–200
[https://doi.org/10.1016/j.ibiod.2012.09.008]
Department of Bioenvironmental Energy, College of Natural Resource and Life Sciences, Pusan National University ichaanisadea@gmail.com
Department of Bioenvironmental Energy, College of Natural Resource and Life Sciences, Pusan National University environ@pusan.ac.kr
Department of Bioenvironmental Energy, College of Natural Resource and Life Sciences, Pusan National University jangsh@pusan.ac.kr
Department of Bioenvironmental Energy, College of Natural Resource and Life Sciences, Pusan National University schong@pusan.ac.kr
Department of Bioenvironmental Energy, College of Natural Resource and Life Sciences, Pusan National University suhjm@pusan.ac.kr
Department of Bioenvironmental Energy, College of Natural Resource and Life Sciences, Pusan National University esjung@pusan.ac.kr
Department of Bioenvironmental Energy, College of Natural Resource and Life Sciences, Pusan National University ssivaphd@pusan.ac.kr