
Algicidal Activity and Stability of a Potential Biological Control Agent Against Harmful Algal Blooms
Ⓒ The Korean Environmental Sciences Society. All rights reserved.
This is an Open-Access article distributed under the terms of the Creative Commons Attribution Non-Commercial License (http://creativecommons.org/licenses/by-nc/3.0) which permits unrestricted non-commercial use, distribution, and reproduction in any medium, provided the original work is properly cited.
Abstract
Harmful algal blooms (HABs) threaten marine ecosystems and require effective control. In this study, Arthrobacter sp. DC-25, an algicidal bacterium, caused a 98% reduction in Chattonella marina cell density. Phylogenetic analysis confirmed its close relationship with Arthrobacter oxydans DSM 20119T. The optimal growth conditions for DC-25 were determined to be 25 °C, pH 7.0, and 2.0% salinity. A algicidal activity correlated with bacterial growth and peaked during the stationary phase. Species-specific effects were observed, with A. catenella and C. marina being highly susceptible, whereas other HAB species showed lower sensitivity. No algicidal effects were detected in non-HAB species. The activity remained stable at 20–50 °C and pH 6–9 but declined at ≥70 °C and pH ≤5. These findings suggest Arthrobacter sp. DC-25 is a promising biological control agent for HABs.
Keywords:
Algicidal bacteria, Arthrobacter, Biocontrol, Chattonella marina, Harmful algal blooms, pH stability, Thermal stability1. Introduction
Phytoplankton are fundamental components of marine ecosystems, serving as primary producers at the base of the aquatic food web (Sarthou et al., 2005). However, under certain environmental conditions, some microalgae proliferate excessively, forming harmful algal blooms (HABs), which can have detrimental effects on marine life, human health, and economic activities (Hallegraeff et al., 2021). Although the term “red tide” is commonly used, HABs can appear in various colors, and not all algal blooms are necessarily harmful. Since its introduction in 1974, the term HABs has been widely adopted to describe blooms that adversely affect ecosystems, irrespective of water discoloration.
The frequency and intensity of HAB events have increased globally, likely driven by climate change, eutrophication, and other anthropogenic influences (Glibert et al., 2005). HABs pose significant threats to fisheries, aquaculture, and public health. Temporal increases in HABs, over the past several decades have been linked to stresses associated with human activities (Vila and Berdalet, 2021), including increased eutrophication and changes in climate that promote blooms of some HAB species (Berdalet et al., 2016). The consumption of seafood contaminated with HAB toxins can lead to severe poisoning syndromes, including paralytic shellfish poisoning (PSP), diarrhetic shellfish poisoning (DSP), and ciguatera fish poisoning (CFP) (Camacho et al., 2007). Moreover, HAB species such as Alexandrium catenella and Chattonella marina are responsible for mass fish mortalities, causing substantial economic losses in fisheries and aquaculture industries (Steidinger, 1983).
HABs are caused by various microalgae, including dinoflagellates, diatoms, cyanobacteria, raphidophytes, and haptophytes (Sournia, 1995). Among these, dinoflagellates of the genus Alexandrium and raphidophytes such as C. marina are of particular concern due to their ecological impact. Alexandrium species are well known for producing paralytic shellfish toxins (PSTs), which accumulate in filter-feeding bivalves and can lead to PSP in humans. The toxicity of Alexandrium species varies among strains, but A. catenella is considered one of the most potent PST producers, frequently causing large-scale shellfish contamination and fish mortality events (Bazzoni et al., 2020). On the other hand, C. marina is responsible for severe fish-killing events worldwide, particularly in aquaculture regions. Unlike Alexandrium, which primarily exerts its toxicity through food-web bioaccumulation, Chattonella species are highly ichthyotoxic, directly affecting fish gill tissues through the production of reactive oxygen species (ROS) (Marshall et al., 2005). Blooms of C. marina have led to catastrophic fish mortalities in Japan and Korea, significantly impacting the aquaculture industry.
The control of HABs remains a major challenge due to their complex and varied causes. In Korea, yellow loess has been used as a control measure, but its application poses risks to non-target marine organisms and may disrupt ecological balance (Park, 2007). In recent years, biological control methods have gained increasing attention as environmentally sustainable alternatives. These include the use of viruses, protozoans, fungi, and bacteria with algicidal properties (Su et al., 2017; Imai et al., 2021; Jeong and Son, 2021; Zhang et al., 2021). Among these, algicidal bacteria have demonstrated significant potential due to their ability to selectively inhibit or kill HAB species while minimizing collateral effects on non-target organisms.
Despite growing interest in biological control strategies, studies on the algicidal potential of Gram-positive bacteria remain limited. This study aims to explore the efficacy of Arthrobacter sp. DC-25 as an algicidal bacterium against HAB-forming species, particularly A. catenella and C. marina. The algicidal activity of Arthrobacter sp. DC-25 was examined, focusing on its specificity and algicidal spectrum against HAB species and live food organisms. Understanding its ecological role will contribute to the development of environmentally friendly strategies for the mitigation of HABs in marine environments.
2. Materials and Methods
2.1. Algal cultures
Alexandrium catenella, Chattonella marina, Cochlodinium polykrikoides, and Heterosigma akashiwowere supplied by the National Fisheries Research & Development Institute (NFRDI), Republic of Korea; Akashiwo sanguinea, Fibriocapsa japonica, Gymnodinium impudicum, Isochrysis galbana, Prorocentrum micans, Prorocentrum minimum, and Scrippsiella trochoideawere obtained from the South Sea Institute of the Korea Ocean Research & Development Institute (KORDI), Republic of Korea; and Chlorella ellipsoidea (C-020), Chlorella vulgaris (C-012), Navicula elegans (B-225), Nannochloris oculata (C-031), Nitzschia pungens (B-037), Oscillatoria angustissima (CY-003), Pavlova gyrans (H-012), Phaeodactylum tricornutum (B-255), Skeletonema costatum (B-687), and Tetraselmis suecica (P-009) were provided by the Korea Marine Microalgae Culture Center, Busan, South Korea.
The dinoflagellate strains (A. sanguinea, A. catenella, C. polykrikoides, F. japonica, G. impudicum, P. micans, P. minimum, and S. trochoidea) and raphidophyte strains (C. marina and H. akashiwo) were maintained in f/2-Si medium (Guillard and Ryther, 1962) at 20°C and pH 8 under a 12 h dark/12 h cool white fluorescent light cycle (120 μmol photons m–2s–1). Strains classified as bacillariophyceae (N. elegans, N. pungens, P. tricornutum, and S. costatum) as well as live food organisms (C. ellipsoidea, C. vulgaris, I. galbana, N. oculata, O. angustissima, P. gyrans, and T. suecica) were maintained in the same f/2-Si medium at 20°C and pH 8, but under a lower light intensity (30 μmol photons m–2s–1).
2.2. Isolation and screening of algicidal bacteria
Water samples were collected from coastal surface waters in Jinhae Bay, South Korea. Samples were serially diluted with sterile seawater, and 0.1 mL aliquots of each dilution were spread onto PPES-II agar plates (Taga, 1968). After incubation at 20°C for seven days, individual colonies exhibiting distinct morphologies were re-streaked onto fresh PPES-II agar plates for purification and subsequently stored at –70°C in 20% glycerol.
The isolated bacteria were precultured in 10 mL of PPES-II broth at 20°C with shaking at 200 rpm for 48 h. For screening purposes, C. marina (1.0 × 104 cells/mL, mid-exponential phase) was used. In each well of a 24-well plate, 1 mL of algal culture was combined with 0.2 mL of bacterial culture (or filtrate or medium control), and the plates were monitored microscopically at ×200 magnification. A bacterial strain exhibiting marked algicidal activity against C. marina was selected for further study.
2.3. Identification of bacterium by phylogenetic analysis
Morphological observations were performed via Gram staining, and conventional biochemical tests were conducted according to the methods described by Smibert and Krieg(1994). Bacterial strain was identified by PCR amplification of the 16S rRNA gene, followed by BLAST analysis against sequences in the GenBank database.
Two oligonucleotide primers, as described by Dunbar et al.(2000), were employed: (Forward) 5'-AGAGTTTGATCCTGGCTCAG-3' and (Reverse) 5'-TACCTTGTTACGACTT-3'. PCR was conducted using intact cells that were heat-treated at 95°C for 5 min as the template. The thermal cycling conditions consisted of 25 cycles of denaturation (1 min at 94°C), annealing (1 min at 55°C), and extension (2 min at 72°C), with a final extension at 72°C for 5 min. The resulting 16S rDNA amplicon was purified, cloned into the pGEM-T Easy vector (Promega, Madison, WI, USA), and sequenced. Sequence comparisons were performed using the BLASTN tool (http://www. ncbi.nlm.nih.gov/BLAST) at the National Center for Biotechnology Information (NCBI). Alignments were generated using CLUSTAL W, and a phylogenetic tree was constructed with MEGA version 7.0 (Kumar et al., 2016).
2.4. Relationship between bacterial growth and algicidal activity
The growth curve and algicidal activity of the DC-25 strain were monitored at 3 h intervals over a 36 h period. The DC-25 strain was inoculated into PPES-II broth (initial cell concentration: 103 cells/mL) and incubated under optimal conditions (25°C, pH 7.0, 2.0% NaCl) with shaking at 200 rpm; optical density (OD) measurements were taken every 3 h. The algicidal activity of the culture filtrate, as well as that of bacterial cells from each growth phase, was evaluated by adding the filtrate to C. marina cultures (1.0 × 104 cells/mL) in a 24-well plate under the same algal culture conditions.
The bioassay plates were incubated under the previously described algal culture conditions, and the number of viable, motile cells in each well was counted using a Sedgewick-Rafter chamber and an inverted microscope. The algicidal activity of DC-25 strain was calculated, using the following equation (Kim et al., 2007): Algicidal activity (%) = (1–Tt/Ct) × 100. Tt (treatment) and Ct (control) are the cell concentrations of C. marina with culture filtrate of DC-25 strain, and sterile PPES‑II broth, respectively, after inoculation time (t).
2.5. Algicidal spectrum of culture filtrate from bacterial DC-25 strain
The culture filtrate of the DC-25 strain was prepared as described above. Its algicidal spectrum was evaluated against a range of harmful algal bloom (HAB) species and live food organisms, including A. sanguinea, A. catenella, C. marina, C. ellipsoidea, C. vulgaris, C. polykrikoides, F. japonica, G. impudicum, H. akashiwo, I. galbana, N. oculata, N. elegans, N. pungens, O. angustissima, P. gyrans, P. tricornutum, P. micans, P. minimum, S. trochoidea, S. costatum, and T. suecica.
For each species at the mid-exponential growth phase (1.0 × 104 cells/mL), 5% (v/v) of the culture filtrate was added. The test flasks were then incubated under the previously described algal culture conditions for 12 h. Algicidal activity was quantified using the established equation.
2.6. Thermal and pH stability of the algicidal activity
The thermal and pH stability of the algicidal activity of Arthrobacter sp. DC-25 were evaluated under various conditions. For thermal stability assessment, culture filtrates from bacterial cultures grown in PPES-II broth at 30°C were incubated at 4, 20, 40, 50, 70, and 100°C for 30 minutes. For pH stability, the filtrates were adjusted using 0.1 M citrate-phosphate buffer (pH 3–7) or 0.1 M Tris-HCl buffer (pH 8–10) and incubated for 30 minutes. Following these treatments, 5% (v/v) of the filtrates was added to C. marina cultures (1.0 × 10⁴ cells/mL) to determine the residual algicidal activity. The relative algicidal activity was expressed as a percentage, with activity at 4°C and pH 7.0 or 8.0 set as 100%. PPES-II broth subjected to the same treatments was used as a control. Data are presented as the mean ± standard deviation from triplicate assays.
3. Results and Discussion
3.1. Screening of algicidal bacteria
A total of 42 bacterial strains were isolated, of which four exhibited algicidal activity against C. marina. The algicidal activity of these four strains was evaluated, and strain DC-25 demonstrated the highest efficacy, reducing C. marina cell density by 98%.
3.2. Identification and characterization of DC-25 strain
To genetically characterize strain DC-25, the 16S rRNA gene was amplified and sequenced, followed by alignment with available sequences in the GenBank database. Sequence analysis revealed that strain DC-25 exhibited high similarity to both Arthrobacter oxydans DSM 20119ᵀ (X83408) and A. polychromogenes DSM 20136ᵀ (X80741), with nearly identical sequence identity (99.2%). Phylogenetic analysis based on 16S rRNA gene sequences confirmed that strain DC-25 belongs to the genus Arthrobacter (Fig. 1). The neighbor-joining tree showed that DC-25 formed a distinct clade, closely related to A. polychromogenes DSM 20136ᵀ with a bootstrap support value of 98%. The strain was positioned within a well-supported cluster that included A. oxydans, A. ureafaciens, and A. ilicis, further supporting its taxonomic placement within the genus.
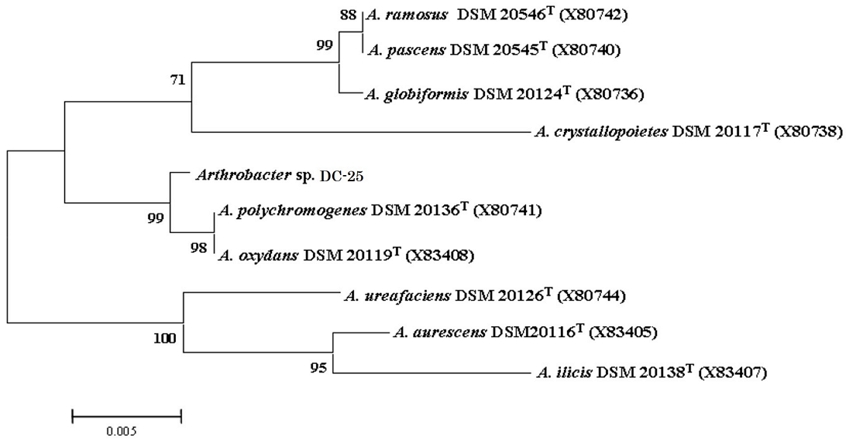
Phylogenetic tree based on 16S rRNA gene sequences of the isolated DC-25 strain and closely related species in the genus Arthrobacter. The numbers at the nodes indicate bootstrap support values (%), derived from neighbor-joining analysis of 1,000 resampled datasets. The scale bar represents 0.005 nucleotide substitutions per site.
Physiological characterization revealed that Arthrobacter sp. DC-25 exhibited optimal growth at 25°C, pH 7.0, and 2.0% salinity (Fig. 2). The strain did not grow below 10°C or at pH values lower than 5.0 but demonstrated tolerance to a range of salinity conditions.
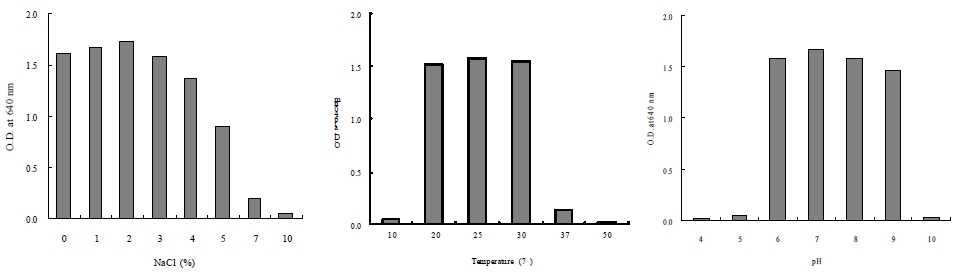
Effects of temperature, initial pH, and NaCl concentrations on the growth of Arthrobacter sp. DC-25 in PPES-II medium.
Although numerous algicidal bacteria have been isolated from various environments, they are predominantly affiliated with the Cytophaga-Flavobacterium-Bacteroidetes (CFB) group or γ-Proteobacteria (Nakashima et al., 2006) or the genus Pseudoalteromonas (Mu et al., 2007a). In contrast, Arthrobacter species, which are Gram-positive bacteria, have been rarely reported to exhibit algicidal activity in marine environments. Previous studies have demonstrated that other Gram-positive genera, such as the genus of Bacillus, can produce algicidal metabolites effective against harmful algae (Mu et al., 2007b; Jeong and Son, 2021). Given that Gram-positive bacteria are typically found in deep-sea sediments rather than in surface waters (Li et al., 1999), their role in algicidal interactions may have ecological significance, potentially functioning as a top-down control mechanism in marine ecosystems (Liu et al., 2008).
The identification of Arthrobacter sp. DC-25 as an algicidal strain expands the understanding of Gram-positive bacteria in algal bloom control. Its genetic and physiological characteristics suggest that it may play a distinct role in regulating harmful algal blooms (HABs), warranting further investigation into its algicidal mechanisms and potential environmental applications.
3.3. Relationship between bacterial growth and algicidal activity of DC-25 strain
Fig. 3 illustrates the growth curve of Arthrobacter sp. DC-25 under optimal culture conditions (25°C, pH 7.0, 2.0% NaCl) and the corresponding algicidal activity of the culture filtrate at different bacterial growth phases against C. marina. The bacterial growth curve (represented by optical density at 640 nm) exhibited a typical sigmoidal trend, with an initial lag phase observed from 0 to 6 h. This was followed by an exponential growth phase from approximately 6 to 18 h, where a sharp increase in bacterial density was detected. The growth rate then gradually slowed, and the stationary phase was reached after 24 h, maintaining a high cell density until the end of the observation period (36 h).
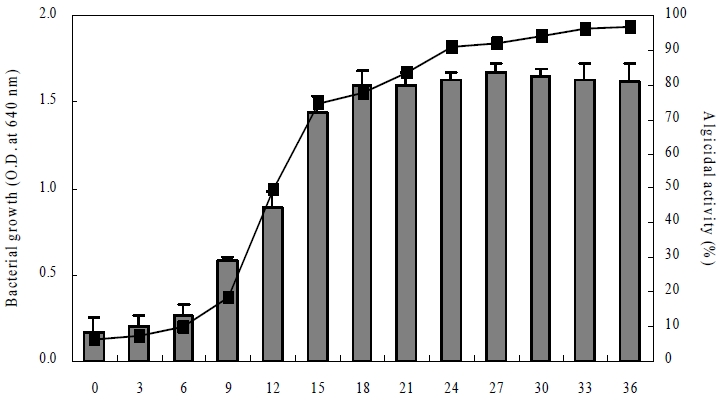
Growth curve of Arthrobacter sp. DC-25 at optimal culture conditions (25°C, pH 7.0, 2.0% NaCl) and algicidal activity by the culture filtrate of each growth phase of Arthrobacter sp. DC-25 against Chattonella marina. -■- = bacterial growth curve (O.D. at 640 nm). Bar graph= algicidal activity (control = algal cultures with PPES-II broth added).
The algicidal activity of the culture filtrate displayed a time-dependent pattern, increasing progressively with bacterial growth. Notably, a significant algicidal effect was first observed at 9 h, coinciding with the early exponential phase of bacterial growth. The algicidal activity continued to increase, peaking at 24 h, which corresponded to the stationary growth phase. Beyond this point, the algicidal activity remained stable, with no substantial decline observed up to 36 h.
The results indicate that Arthrobacter sp. DC-25 exerts its algicidal effect through an indirect mechanism, releasing algicides into the surrounding environment. Indirect algicidal mechanisms, observed in approximately 70% of algicidal bacteria (Mayali and Azam, 2004; Roth et al., 2008), provide a competitive advantage by allowing bacteria to target a broad range of harmful algal species without direct interaction. Consistent with previous studies demonstrating a correlation between bacterial cell density and algicidal activity (Nakashima et al., 2006; Mu et al., 2007b), Arthrobacter sp. DC-25 exhibited its highest algicidal activity during the late exponential and early stationary phases. This suggests that the algicidal compounds produced by this strain are likely secondary metabolites synthesized in the later stages of growth. The sustained high algicidal effect during the stationary phase further implies that these compounds are either continuously produced or remain stable in the culture filtrate over time. Further research is needed to elucidate their chemical nature and stability.
3.4. Algicidal spectrum of culture filtrate from DC-25 against HAB species
The algicidal spectrum of culture filtrate from Arthrobacter sp. DC-25 against various harmful algal bloom (HAB)-forming species was evaluated, and the results are presented in Fig. 4. The data indicate that the algicidal activity of Arthrobacter sp. DC-25 varies significantly depending on the target algal species.
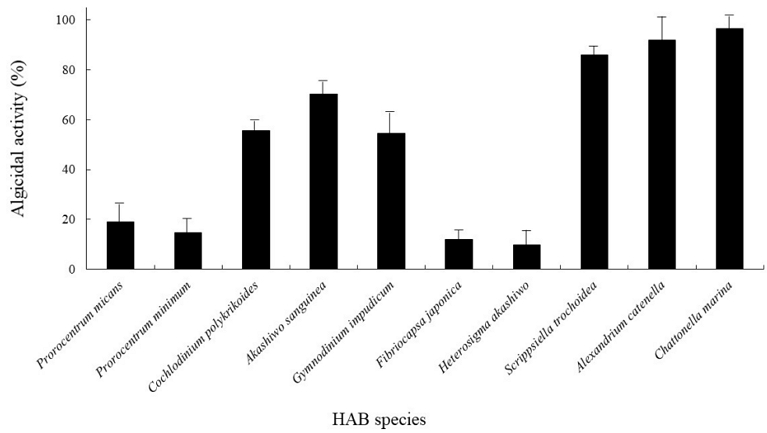
Algicidal spectrum of Arthrobacter sp. DC-25 against various HAB-forming species. The algicidal activity of Arthrobacter sp. DC-25 was assessed against multiple harmful algal bloom (HAB)-forming species. A 5% culture filtrate was introduced into each algal culture at the mid-exponential growth phase, and algicidal activity was evaluated after 12 h. The control group consisted of algal cultures supplemented with sterile PPES-II broth. Data are presented as mean ± standard deviation, based on triplicate assays.
Among the tested species, A. catenella and C. marina exhibited the highest susceptibility to Arthrobacter sp. DC-25, with algicidal activity exceeding 98%. This suggests that Arthrobacter sp. DC-25 produces bioactive compounds or induces physiological stress that effectively suppresses these algal species. Similarly, A. sanguinea and G. impudicum showed moderate levels of algicidal activity, ranging from 50% to 70%, indicating a potential species-specific mode of action. In contrast, F. japonica, H. akashiwo, and S. trochoidea exhibited relatively low susceptibility to Arthrobacter sp. DC-25, with algicidal activity below 20%. The Prorocentrum species (P. micans and P. minimum) and C. polykrikoides also demonstrated minimal algicidal responses, suggesting either resistance to the bacterial metabolites or limited interaction between Arthrobacter sp. DC-25 and these algal species.

Algicidal effects of Arthrobacter sp. DC-25 on the growth of Bacillariophyceae and live food organisms
The differential susceptibility among HAB species may be attributed to variations in cell wall composition, physiological defense mechanisms, or differential responses to bacterial-secreted compounds. Previous studies have shown that algicidal bacteria can exhibit species-specific activity through the production of bioactive compounds. For example, Umetsu et al.(2019) reported that Alteromonas sp. produced the algicidal compound Questiomycin A, which effectively inhibited the growth of C. antiqua. Similarly, the strong algicidal effect of Arthrobacter sp. DC-25 against A. catenella and C. marina suggests the presence of potent bioactive metabolites targeting these HAB species.
These findings highlight the potential of Arthrobacter sp. DC-25 as a candidate for HAB mitigation strategies. Future investigations should focus on identifying the specific algicidal compounds produced by Arthrobacter sp. DC-25 and elucidating the molecular mechanisms underlying its selective activity. Furthermore, additional studies should assess its effectiveness under natural environmental conditions and evaluate any potential ecological risks associated with its application in marine ecosystems.
3.5. Algicidal effects of culture filtrate from DC-25 on the growth of Bacillariophyceae and live food organisms
The algicidal effects of the culture filtrate from Arthrobacter sp. DC-25 were assessed on various algal species, including Bacillariophyceae, Chlorophyceae, Cyanophyceae, Haptophyceae, and Prasinophyceae. The results indicated that Arthrobacter sp. DC-25 did not exhibit algicidal activity against any of the tested species, as all results were negative. Notably, among Bacillariophyceae, N. pungens, a known harmful algal bloom (HAB)-forming species, showed an increase in cell density, suggesting a potential stimulatory effect rather than inhibition, while other HAB-associated species, such as S. costatum, remained unaffected. Similarly, no algicidal effects were observed in Chlorophyceae (C. ellipsoidea, C. vulgaris, and N. oculata), Cyanophyceae (O. angustissima), Haptophyceae (I. galbana and P. gyrans), or Prasinophyceae (T. suecica).
Although indirectly acting algicidal bacteria often exhibit species-specific effects mediated by chemical compounds (Yoshinaga et al., 1997; Jeong and Son, 2021), Arthrobacter sp. DC-25 did not display strict species specificity but instead demonstrated a broad host range. While no algicidal effects were observed on Bacillariophyceae and live food organisms, the strain exhibited strong algicidal activity against A. catenella and C. marina, with no adverse effects on beneficial marine species. The mechanism underlying this selective activity may be associated with bacterial secondary metabolites that disrupt algal cell integrity. For example, mycosubtilins produced by bacteria in the genus Bacillus interact with the cytoplasmic membrane, resulting in increased ion permeability and lysis of both dinoflagellate and raphidophyte species (Jeong and Son, 2021). Similarly, the strong activity of Arthrobacter sp. DC-25 against A. catenella and C. marina suggests that it may produce bioactive compounds with a mode of action distinct from those affecting Bacillariophyceae.
This selective algicidal activity highlights the potential of Arthrobacter sp. DC-25 as a targeted biological control agent for HABs. Further investigations are needed to determine whether different environmental conditions or bacterial metabolites could influence its algicidal potential, as well as to identify the specific bioactive compounds responsible for its selective effects.
3.6. Thermal and pH stability of the algicidal activity of Arthrobacter sp. DC-25
The thermal and pH stability of the algicidal compounds produced by Arthrobacter sp. DC-25 were evaluated under different conditions (Table 2). For thermal stability, the culture filtrate was incubated at 4, 20, 40, 50, 70, and 100°C for 30 minutes before activity measurement. For pH stability, the filtrate was adjusted to pH 3–10 using appropriate buffers and incubated for 30 minutes prior to assessment.
The results indicate that the algicidal activity of Arthrobacter sp. DC-25 remained relatively stable at moderate temperatures (20–50°C), with only a slight reduction observed at elevated temperatures (70°C: 87.0 ± 7.4%; 100°C: 78.0 ± 9.2%). In terms of pH stability, the algicidal activity remained high in neutral to slightly alkaline conditions (pH 6–9) but declined significantly under more acidic conditions (pH 3–5), with the lowest activity recorded at pH 3.0 (34.6 ± 11.6%).
These findings suggest that Arthrobacter sp. DC-25 exhibits strong thermal stability within a moderate temperature range and maintains optimal algicidal activity under neutral to slightly alkaline conditions. Given that the average pH and temperature of seawater are approximately 8 and 5°C, respectively, the pH stability and heat tolerance of Arthrobacter sp. DC-25 may provide an ecological advantage in harmful algal bloom (HAB) management.
Unlike chemical control methods, which can negatively impact non-target organisms and disrupt ecosystems, algicidal bacteria offer a more sustainable and environmentally friendly alternative. Arthrobacter sp. DC-25, in particular, demonstrates strong algicidal activity while exhibiting minimal toxicity toward beneficial marine species. These characteristics highlight its potential as a biological control agent for mitigating HABs.
Further research is necessary to identify and characterize the specific algicidal compounds responsible for the observed effects. Understanding their molecular mechanisms, stability, and mode of action will facilitate the development of optimized application strategies for HAB mitigation. Additionally, large-scale field studies are required to assess the efficacy and ecological impact of introducing Arthrobacter sp. DC-25 into natural marine environments. With continued investigation, algicidal bacteria such as Arthrobacter sp. DC-25 could become a key component of integrated HAB management strategies, offering an effective and ecologically sustainable solution to harmful algal blooms.
4. Conclusions
This study identified and characterized the algicidal bacterium Arthrobacter sp. DC-25, which demonstrated potent algicidal activity against C. marina and several other harmful algal bloom (HAB)-forming species. Among the 42 isolated bacterial strains, DC-25 exhibited the highest algicidal efficiency, achieving a 98% reduction in C. marina cell density. Molecular identification based on 16S rRNA gene sequencing confirmed that DC-25 belongs to the genus Arthrobacter, with the highest sequence similarity (99.2%) to Arthrobacter oxydans DSM 20119T. The optimal growth conditions for Arthrobacter sp. DC-25 were determined to be 25°C, pH 7.0, and a salinity of 2.0%.
The algicidal activity of DC-25 was closely associated with its growth phase, with the highest activity observed during the stationary phase. This suggests that algicidal compounds are actively produced and/or accumulate in the culture filtrate over time. The algicidal spectrum analysis revealed a species-specific effect, with A. catenella and C. marina being the most susceptible, while other HAB-forming species, such as P. micans and C. polykrikoides, exhibited resistance. Notably, the culture filtrate had no algicidal effects on Bacillariophyceae, Chlorophyceae, Cyanophyceae, Haptophyceae, or Prasinophyceae, indicating its specificity toward certain HAB species.
Furthermore, the algicidal activity of Arthrobacter sp. DC-25 was found to be stable under a wide range of environmental conditions. The activity remained relatively high at moderate temperatures (20–50°C) and in neutral to slightly alkaline pH conditions (pH 6–9). However, significant reductions in algicidal efficiency were observed at extreme temperatures (≥70°C) and under highly acidic conditions (pH 3–5). These findings suggest that the algicidal compounds produced by Arthrobacter sp. DC-25 are relatively stable under environmentally relevant conditions, making them promising candidates for HAB mitigation strategies.
Overall, this study provides important insights into the potential use of Arthrobacter sp. DC-25 as a biological control agent against HABs. Its strong algicidal activity, species-specific effects, and stability under moderate environmental conditions highlight its applicability for HAB management. Future studies should focus on identifying the specific algicidal compounds involved, elucidating their mechanisms of action, and assessing their ecological safety in natural environments. The integration of Arthrobacter sp. DC-25 into HAB control strategies may offer an effective and environmentally sustainable approach to mitigating the impacts of harmful algal blooms in marine ecosystems.
Acknowledgments
This work was supported by the sabbatical research grant from Daegu Catholic University in 2022.
REFERENCES
-
Bazzoni, A. M., Cangini, M., Mudadu, A. G., Lorenzoni, G., Arras, I., Sanna, G., 2020, Recent findings of paralytic shellfish toxins linked to the genus Alexandrium Halim in Mediterranean mollusc production areas, Toxicon, 174, 48–56.
[https://doi.org/10.1016/j.toxicon.2019.12.157]
-
Berdalet, E., Fleming, L. E., Gowen, R., Davidson, K., Hess, P., Backer, L. C., 2016, Marine harmful algal blooms, human health and wellbeing: Challenges and opportunities in the 21st century, J. Mar. Biol. Assoc., 96, 61–91.
[https://doi.org/10.1017/S0025315415001733]
-
Camacho, F. G., Rodríguez, J. G., Mirón, A. S., García, M. C. C., Belarbi, E. H., Chisti, Y., Grima, E. M., 2007, Biotechnological significance of toxic marine dinoflagellates, Biotechnol. Advan., 25, 176–194.
[https://doi.org/10.1016/j.biotechadv.2006.11.008]
-
Dunbar, J., Ticknor, L. O., Kuske, C. R., 2000, Assessment of microbial diversity in four Southwestern United States soils by 16S rRNA gene terminal restriction fragment analysis, Appl. Environ. Microbiol., 66, 2943–2950.
[https://doi.org/10.1128/AEM.66.7.2943-2950.2000]
-
Glibert, P. M., Anderson, D. M., Gentien, P., Granéli, E., Sellner, K. G., 2005, The global complex phenomena of harmful algal blooms. Oceanography, 18, 136–147.
[https://doi.org/10.5670/oceanog.2005.49]
-
Guillard, R. R. L., Ryther, J. H., 1962, Studies of marine planktonic diatoms. 1. Cyclotella nana (HUSTEDT) and Detonula confervacea (cleve) Gran, Can. J. Microbiol., 8, 229–239.
[https://doi.org/10.1139/m62-029]
-
Hallegraeff, G. M., Anderson, D. M., Belin, C., Bottein, M. Y. D., Bresnan, E., Chinain, M., 2021, Perceived global increase in algal blooms is attributable to intensified monitoring and emerging bloom impacts, Commun. Earth Environ., 2, 117.
[https://doi.org/10.1038/s43247-021-00178-8]
-
Imai, I., Inaba, N., Yamamoto, K., 2021, Harmful algal blooms and environmentally friendly control strategies in Japan, Fish. Sci., 87, 437–464.
[https://doi.org/10.1007/s12562-021-01524-7]
-
Jeong, S. Y., Son, H. J., 2021, Effects of mycosubtilin homolog algicides from a marine bacterium.Bacillus sp. SY-1, against the harmful algal bloom species Cochlodinium polykrikoides, J. Microbiol., 59, 389–400.
[https://doi.org/10.1007/s12275-021-1086-8]
-
Kim, J. D., Kim, B., Lee, C. G., 2007, Alga-lytic of Pseudomonas fluorescens against the red tide causing marine alga Heterosigma akshiwo (Raphidophyceae), Biologic. Control, 41, 296–303.
[https://doi.org/10.1016/j.biocontrol.2007.02.010]
-
Kumar, S., Stecher, G., Tamura, K., 2016, MEGA7: molecular evolutionary genetics analysis version 7.0 for bigger datasets, Mol. Biol. Evol., 33, 1870–1874.
[https://doi.org/10.1093/molbev/msw054]
- Li, H., Yu, Y., Luo, W., 1999, Distribution and diversity of heterotrophic bacteria in deep-sea sediments, Acta. Oceanologica. Sinica., 18, 537-544.
- Liu, J., Fu, B., Yang, H., Zhao, M., He, B., Zhang, X. H., He, Y., 2008, Phylogenetic diversity, composition structure, and distribution patterns of bacterial communities in surface sediments of the Bohai Sea and the Yellow Sea, Appl. Microbiol. Biotechnol., 80, 625-638.
-
Marshall, J. A., Ross, T., Pyecroft, S., Hallegraeff, G., 2005, Superoxide production by marine microalgae: II. Towards understanding ecological consequences and possible functions, Mar. Biol., 147, 541-549.
[https://doi.org/10.1007/s00227-005-1597-6]
-
Mayali, X., Azam, F., 2004, Algicidal bacteria in the sea and their impact on algal blooms, J. Eukaryot. Microbiol., 51, 139–144.
[https://doi.org/10.1111/j.1550-7408.2004.tb00538.x]
- Mu, J., Hirayama, K., Nikaido, H., 2007a, Isolation and characterization of a novel marine algicidal bcterium Pseudoalteromonas sp. strain A28, Appl. Environ. Microbiol., 73, 3537-3545.
-
Mu, R. M., Fan, Z. Q., Pei, H. Y., Yuan, X. L., Liu, S. X., Wang, X. R., 2007b, Isolation and algae-lysing characteristics of the algicidal bacterium B5, J. Environ. Sci., 19, 1336–1340.
[https://doi.org/10.1016/S1001-0742(07)60218-6]
-
Nakashima, T., Miyazaki, Y., Matsuyama, Y., Muraoka, W., Yamaguchi, K., Oda, T., 2006, Producing mechanism of an algicidal compound against red tide phytoplankton in a marine bacterium γ-Proteobacterium, Appl. Microbiol. Biotechnol., 73, 684–690.
[https://doi.org/10.1007/s00253-006-0507-2]
-
Park, C. H., 2007, Additive materials to reduce the amount of loess being applied for red tide removal on coastal water, J. Environ. Sci., 16, 745–750.
[https://doi.org/10.5322/JES.2007.16.6.745]
-
Roth, P. B., Twiner, M. J., Mikulski, C. M., Barnhorst, A. B., Doucette, G. J., 2008, Comparative analysis of two algicidal bacteria active against the red tide dinoflagellate Karenia brevis, Harmful Algae, 7, 682–691.
[https://doi.org/10.1016/j.hal.2008.02.002]
-
Sarthou, G., Timmermans, K. R., Blain, S., Tréguer, P., 2005, Growth physiology and fate of diatoms in the ocean: A Review, J. Sea Res., 53, 25–42.
[https://doi.org/10.1016/j.seares.2004.01.007]
- Smibert, R. M., Krieg, N. R., 1994, Phenotypic characterization, in: Methods for general and molecular bacteriology, American Soc. Microbiol., Washington, D. C., U.S.A., 607–654.
- Sournia, A., 1995, Red tide and toxic marine phytoplankton of the world ocean: An Inquiry into biodiversity, In: Lassus, P., Arzul, G., Erard, E., Gentien, P., Marcaillou, C. (eds.), Harmful marine algal blooms, Lavoisier Science Publ., Paris, France, 103–120.
- Steidinger, K. A., 1983, A Re-evaluation of toxic dinoflagellate biology and ecology, Prog. Phycol. Res., 2, 147–188.
-
Su, J. F., Liang, D. H., Huang, T. L., Wei, L., Ma, M., Lu, J. S., 2017, Enhancement of simultaneous algicidal and denitrification of immobilized Acinetobacter sp. J25 with magnetic Fe3O4 nanoparticles, Environ. Sci. Pollution Res., 24, 17853–17860.
[https://doi.org/10.1007/s11356-017-9380-z]
- Taga, N., 1968, Some ecological aspects of marine bacteria in the Kuroshio current, Bull. Misaki Mar. Biol, 12, 65–76.
-
Umetsu, S., Kanda, M., Imai, I., Sakai, R., Fujita, M. J., 2019, Questiomycins, algicidal compounds produced by the marine bacterium Alteromonas sp. D and their production cue, Molecules, 24, 4522.
[https://doi.org/10.3390/molecules24244522]
-
Vila, M., Camp, J., Berdalet, E., 2021, Toxic microalgae and global change: Why have proliferations increased along the Mediterranean coast?, Metode, 2021, 201–207.
[https://doi.org/10.7203/metode.11.17108]
-
Yoshinaga, I., Kawai, T., Ishida, Y., 1997, Analysis of algicidal ranges of the bacteria killing the marine dinoflagellate Gymnodinium mikimotoi isolated from Tanabe Bay, Wakayama Pref., Japan, Fish. Sci., 63, 94–98.
[https://doi.org/10.2331/fishsci.63.94]
-
Zhang, Y. L., Chen, D., Zhang, N., Li, F., Luo, X. X., Li, Q. R., 2021, Transcriptional analysis of Microcystis aeruginosa co-cultured with algicidal bacteria Brevibacillus laterosporus, Int. J. Environ. Res. Public Health, 18, 8615.
[https://doi.org/10.3390/ijerph18168615]
Department of Biomedical, Daegu Catholic Universityjsymicro@cu.ac.kr
Department of Biomedical, Daegu Catholic Universityivorikim@korea.kr